Nanoscale electro-optomechanical transducers
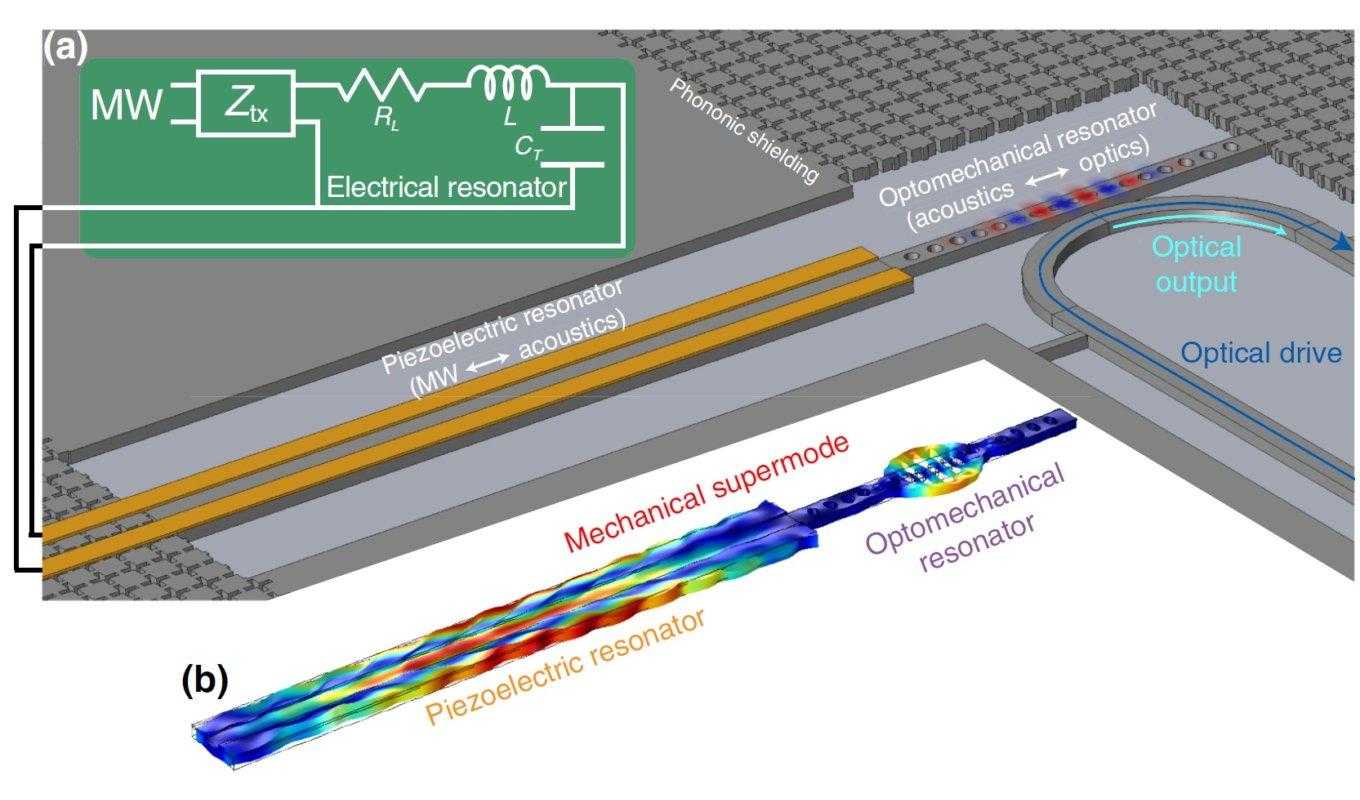
Proposed microwave-to-optical quantum transducer based on a coupled piezoelectric and optomechanical resonator system (Wu et al, Phys Rev. Applied, 2020).
Recent developments in the field of cavity optomechanics have resulted in devices for which GHz frequency phonons and 200 THz frequency optical photons are spatially co-located to the same length scale, often times in the context of structures that simultaneously take advantage of photonic and phononic bandgap concepts. Taken together with the strong photoelastic properties (i.e., the coupling of strain to refractive index) of materials like GaAs, this results in very large optomechanical coupling rates, so that even the phonon's zero-point motion can appreciably shift the frequency of the resonant optical mode confining the photons (Balram et al, Optica 2014). Our work over the past few years has largely been focused on the applications of these GHz mechanical frequency cavity optomechanical devices in signal transduction and sensing.
One application of such high mechanical frequency devices is in thermometry, where under the assumption that the mechanical mode of interest is thermalized to its surroundings, its phonon occupancy gives a measurement of temperature. When probing the mechanical resonator using a laser, however, the resulting readout signal contains signatures of both the mechanical resonator's Brownian motion and its motion due to the fluctuating radiation pressure force associated with shot noise on the probe laser. This quantum back-action effect essentially provides a calibration ruler through which thermal Brownian motion can be gauged, yielding a type of thermometer that can, in principle, ultimately operate over a wide range of wavelengths and act as a primary device (Purdy et al, Science, 2017).
We have also been interested in connecting cavity optomechanical devices to microwave frequency electrical circuits. Potentially, such systems can enable the efficient and low-noise frequency conversion of single microwave photons and single optical photons, of relevance to distributed quantum computing, for example. Our approach has been to utilize GaAs-based optomechanical devices that already reach a regime in which efficeint interconversion of single optical photoncs and single acoustic phonons is possible, and understand how to best embed them within a microwave circuit. A first attempt was in using an interdigitated transducer to create GHz frequency acoustic waves (resonant with the applied microwave field) via the piezoelectric effect. These acoustic waves then load the optomechanical resonator, and we showed that such devices can realize coherent interference effects in either the optical acoustic domains (Balram et al, Nature Photonics, 2016). More recently, our collaborators at TU Delft have shown that such geometries, when operated in a T<100 mK dilution refrigerator, can remain in the quantum ground state of mechanical motion during operation (Forsch et al, Nature Physics, 2019; see also Ramp et al, Physical Review Letters, 2019). Unfortunately, such devices have suffered from very low overall conversion efficiency. Recently (Wu et al, Physical Review Applied, 2020), we have proposed new piezo-optomechanical systems in which this conversion efficiency can be made much more efficient.
Finally, we have also considered novel cavity optomechanical geometries based on slot-mode configuraitons (Grutter et al, Optica, 2015 and Grutter et al, APL Photonics, 2018), which can flexibly realize multi-mode optomechanical configurations with optimized coupling as well as integration of nanoelectromechanical control for tuning, feedback stabilization, and coupling to microwave circuits.
We have been fortunate to collaborate with TU Delft, the University of Alberta, KIST, the University of Copenhagen, NIST, UMD, and the University of Pittsburgh on this work.
Selected representative publications from our recent work are included below; a full list can be found on the Publications page.